Anthia Maysidue is from the Department of Chemical Engineering and Biotechnology and a member of the BioElectronic System Technology Group. Here, we are sharing her virtual lecture for Cambridge Festival 2021, on ‘Organs-on-Chips.’ Visit here.
That is why we call these chips home away from home. Once settled down, these cells continuously feed on sugars and other nutrients dissolved in the liquids pumped in the channels. These channels are next to the cell chambers, so in this way, we mimic how blood is flowing through capillaries surrounding cells on an organ-on-chip. The nutrients can squeeze through tiny pores between the channels because of this membrane that we mentioned before. But the cells cannot escape the chamber. Similarly, the flow can transport waste products produced by the cells and other messenger substances away from this chamber.
Finally, because the material that chips are made up of is transparent in most cases, the cells can be viewed with a microscope through a tiny window at the bottom of the chip.
So, this is how it works, and the benefits of this technology, and how its adoption has a genuine impact on not only the drug discovery field but a wide range of research fields is amazing. Research institutes and the pharmaceutical industry highly value this. The organ-on-chip community is going fast. We now have numerous chips to mimic specific organs and specific functional units. There are numerous ways we can apply these organ-on-chips. There are a few examples. Let us discuss a lung-on-a-chip model.
The first step is to understand the anatomy of the target organ and reduce it to the basic elements essential for physiological function, what we called before functional units. The next step is to examine the different cell types, how they organize and what organ-specific signals are necessary for performing the function. Here, the alveolar sac of the lung consists of alveolar epithelial cells type 1 and vascular epithelial cells type 2 that are closely opposed to each other, and a thin layer of connective tissue separates them. Now, the epithelial and endothelial layers are subjected to air and blood flow, respectively. The interface between the layers experiences mechanical forces induced by breathing motions. This is the organ-specific microenvironment. Now that we have identified the functional unit features and the structural organization, we have to design the chip device accordingly.
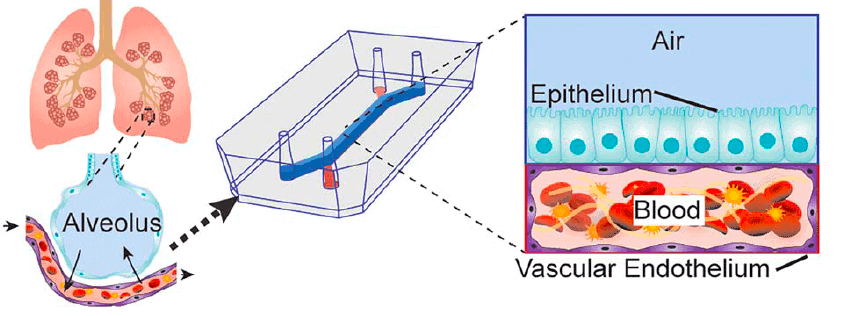
This lung-on-a-chip system consists of two overlapping microchannels that sandwich an inflexible membrane and have tiny pores to enable communication between these two cell types. This design nicely mimics the structure of the alveolar sac, and at the same time, we can co-culture these two types on either side of the membrane. In addition to that, the cells are exposed to their tissue-specific natural environments, so air is pumped into the chambers of the epithelial cells, and fluid mimicking the blood flow is pumped through the endothelial side. We also said that the alveolar sac experiences mechanical forces as a result of breathing. To mimic that, the device is fitted with two hollow microchambers alongside the culture channels that we can mechanically stretch. The stretching of the membranes containing both types is mimicking breathing mainly.
Another example is the blood-brain barrier-on-a-chip, BBB-on-a-chip. It is a unique and highly specialized tissue that acts as a selective barrier that controls the transport of molecules from our blood to our brain. And it also maintains physiological conditions for optimal function of our CNS. The BBB is composed of several cell types that collaborate to form this tight barrier. Along with the extracellular matrix (a gel-like tissue that lies between our organs) and ECM, these BBB cells create a microenvironment optimum for function. Neither animal models of the BBB nor conventional cell cultures in a dish accurately mimic the BBB, or the transport functions of the BBB observed in our body. That is why there is a great need for a BBB model to develop new and effective therapeutics so that they can be safely administered to the brain and have these models as tools to deepen our understanding of disease mechanisms.
Researchers use devices comprised of two-chamber separated by a thin, flexible membrane. One side of the membrane is lined with brain epithelial cells; the other chamber is lined with specialized astrocytes and pericytes. Relevant fluids are pumped through each chamber. What was really cool in this example is that the researchers also managed to control the oxygen levels we have in the body. This fact was shown to significantly improve the morphology and the function of this BBB model. Then they used these chips to validate the transport mechanisms of these drugs and other therapeutic molecules across the BBB. In particular, they investigated a series of transport mechanisms that prevent drugs from reaching their target to the brain. Hence, they pump them back into the bloodstream, or in contrast, they allow selective transport of nutrients and drugs across to the brain. They will help a lot of patients with neurodegenerative and neurological disorders.
Another example of organ-on-chips applications is modeling cancer. Here. I’ll discuss pancreatic cancer, which is one of the most contagious forms of the disease. I think an average of only ten percent of the patients is alive a few years after diagnosis. One of the reasons for such a dismal outcome is that pancreatic cancer cells can escape from tumors and enter the bloodstream very early in the disease. This means that by the time the cancer is discovered, it has already spread. Paradoxically, pancreatic tumors almost plague blood vessels altogether, which prevent cancer drugs from reaching and killing those tumors.
This has been puzzling scientists and clinicians a lot who are trying to understand how the disease progresses.
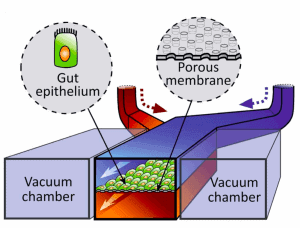
In this device, we have a clear, flexible plastic chip the size of a USB stick again. The chip, in this case, is filled with collagen, and here we have two microfluidic channels, each of which can be linked with living cells that are kept alive with a constant flow of the nutrient-rich medium. One channel mimics the blood vessel, and the other is a pancreatic tumor. What we researchers found out with this device is that tumor cells invade nearby vessels, destroy the epithelial cells lining them and replace those two more lined structures. Besides, the group identified with this chip the interaction of specific protein of pancreatic cancer cells with a receptor on the surface of the endothelial cells as the driving mechanism for cancer metastasis.
This was very surprising, and they suggest that this can use to design future treatments. For example, if we have a molecule that inhibits this interaction, then we could rescue the blood vessels from cancer invasion, and we could deliver drugs that can shrink the tumor mass, which is currently impossible. This organ-on-chip application really exemplifies the power of this technology to replicate the cis-state in the lab, to identify drug targets, and identify mechanisms of drug interaction in a way that is superior to preclinical research.
Let me now show you an application developed by our group; the bio-electronic systems technologies. We use organic polymers, which are electroactive. So, we use these materials as the building blocks to design various devices. Some of these work as organ-on-chips, and we use these materials to interface them with biological systems that are also developed in the lab. Now. As I said, this material is electroactive. This means we can use this material as an integrated cell center.
Basically, when cells grow on the material, they change the properties of the material, by monitoring these electrical properties of the material, we obtain information relevant to the activity of the cells. Whether they are dying or functional, healthy or not, or are they becoming the tissue we want them to become. This is very useful for building organ-on-chips because it enables us to get real-time and continuous information about how cells behave in the chip.
An example of that is our bio-electronic gut-on-a-chip. Essentially, this organ is a cube that has very thick walls of multiple layers and it is the gatekeeper of our body. It is making sure that vitamins, minerals and other essential molecules for our health enter our body. At the same time, it keeps out pathogens and toxins, as well as the microorganisms that reside on the surface of the gut lumen, also known as the microbiome.
Read Part one here: https://scientiamag.org/your-body-on-a-chip%e2%88%92part-one/
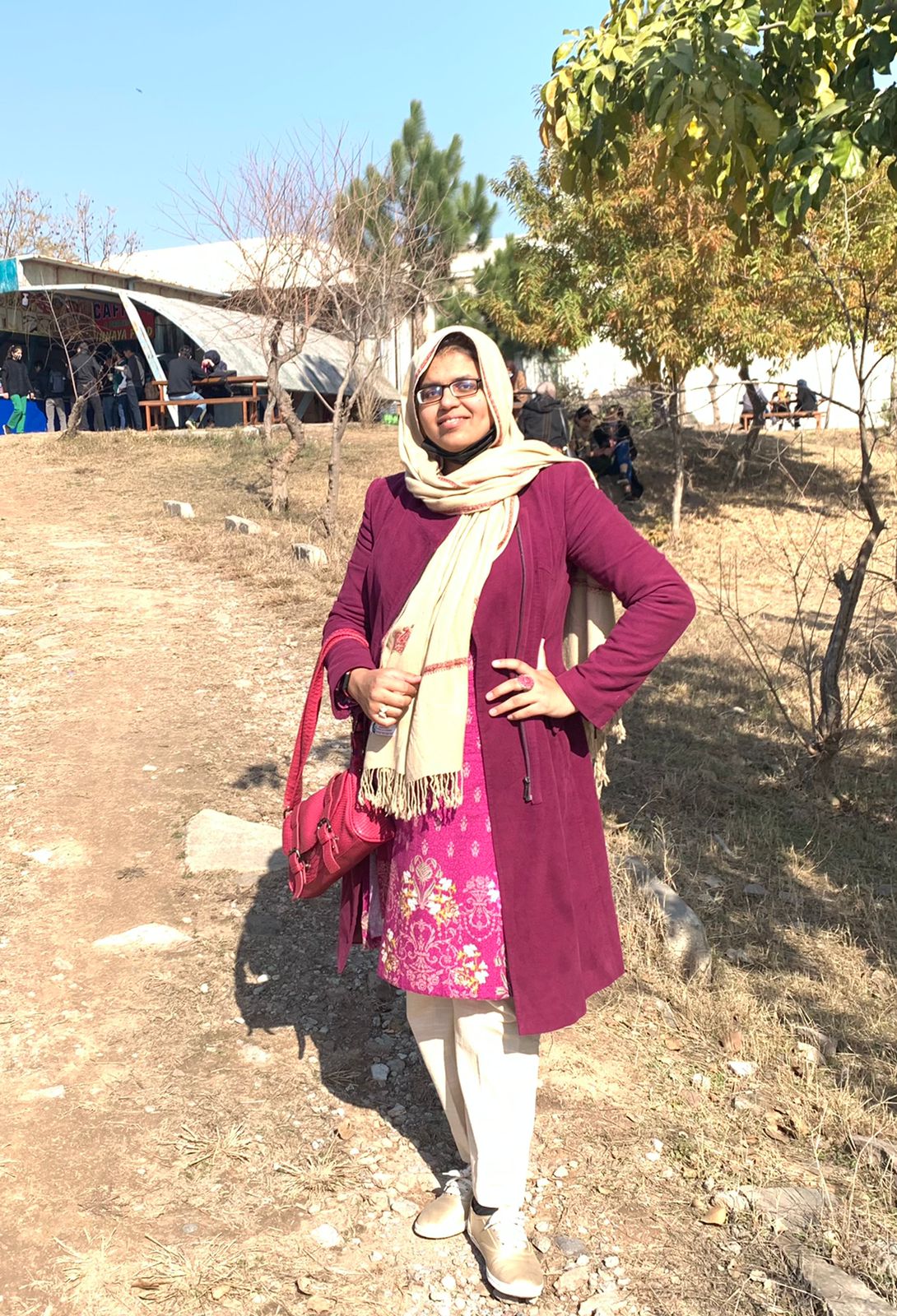
Aniqa Mazhar is a graduate of QAU in Biochemistry. She has taught sciences to O levels and is currently planning for her MS in Food Technology. Aniqa’s hobbies are reading, watching movies, writing, calligraphy, long walks, and nature photography.