This year, we are witnessing the most advanced era of “Infrared Astronomy” in the history of mankind. As we catch sight of observations from the revolutionary James Webb Space Telescope (JWST), astronomers and the general public know more about the universe than ever. This brings us to recall, the very first invention of the “First Optical Telescope”, recorded in the early 1600s, observational astronomy has evolved over a long era. From the early 20th century, the larger optical telescope started to exist on the ground as well as in space.
Later, in the 1970s, rocket-borne or ultraviolet, X-ray and gamma-ray detectors helped us to detect the highest possible energy phenomenon occurring in the different regions of the universe. With this improvement in optical engineering, astronomers were finally able to observe the universe in Infrared, X-ray and other wavelengths of the electromagnetic spectrum, the entire wide array of waves that come in electromagnetic radiation.
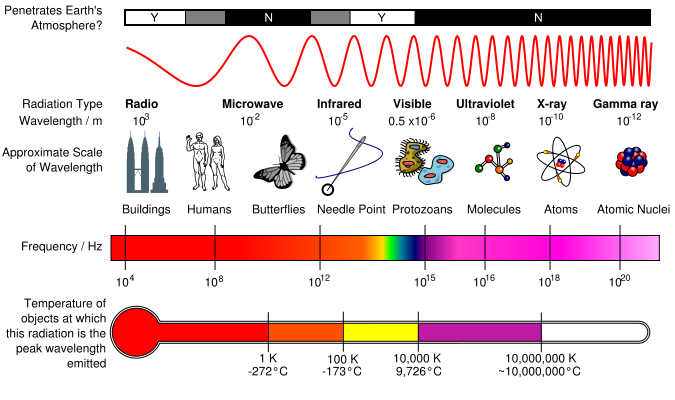
To understand what astronomical phenomenon or what astrophysical event is happening, we try to look at the universe in the multi-wavelength. Since then, when it comes to telescopes, whenever a new window in the electromagnetic spectrum opens for humans to observe, new scientific discoveries are made. As new generations of telescopes are huge and better than previous ones, the basic science and design behind observing the same wavelength have remained the same.
In this short article, we will be discussing each band of the electromagnetic spectrum, from Radio Waves to Gamma Rays, in the context of Astronomical questions. What systems in Astronomy emit this radiation? What physical procedure is occurring in that region? How do we observe this radiation? What are the missions that probe in this wavelength to observe the universe?
RADIO WAVES
The wavelength in the RADIO segment of the spectrum is measured in waves longer than 1 mm and frequency lower than 300 GHz. Radio waves are the lowest emitted energy in the universe, the light is commonly made by a phenomenon of physics called synchrotron radiation, due to the gyration of charged particles around magnetic field lines and free-free radiation, as the charged particles in the electric field decelerate. Mostly, radio waves are emitted in astrophysical phenomena, where we trace magnetic fields and where the particles seem to be accelerating.
If we look for the Active Galactic Nuclei (AGNs) and gamma-ray bursts (GRBs), the supernovas, and tidal disruption events. At very lower luminosity (i-e lower brightness), radio waves can commonly be seen in ionized gas around young, hot OB stars, or giant elliptical galaxies, many of them were discovered in one of the most advanced surveys of our time such EMU – that is expected to detect some 70 million galaxies, known to be the first radio survey of this scale.
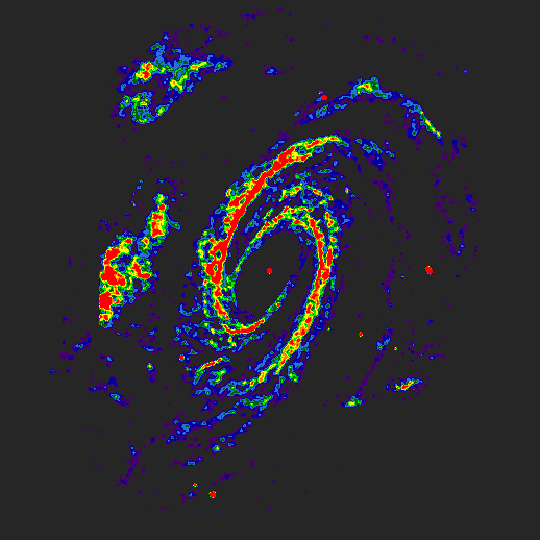
On earth, radio waves are found nearly everywhere, penetrating the clouds, and our communication systems. It’s found to be operated in two ways, first – a huge single radio dish such as a Five Hundred-Meter Aperture Spherical Telescope (FAST) or a whole array of dishes, such as a Very Large Array (VLA), Square Kilometer Array (SKA), or ASKAP – Australian SKA Pathfinder, which was used in the above mentioned EMU Survey, all the arrays are combined together to form one big telescope for a superior resolution using the interferometry method.
MICROWAVE/SUB-MILLIMETER
From 300 microns (μm) to 1 mm with 1 THz to 300 GHz, we classify the part of the spectrum as Microwave or Sub-millimeter, this band lies in between the radio and far-infrared emissions, In astrophysics, the processes that emit microwave or sub-mm can be found in radio wave emitting objects, and thermal emission from cold material can produce light in this spectrum part. The most known and widely discovered phenomenon is the Cosmic Microwave Background (CMB), the earliest light we can observe from the big bang – the creation of the universe.
From our perspective of temperature units, the CMB has a consistent average temperature of 2.725K, with few fluctuations. It can also be seen in higher energy phenomena, such as relativistic jets – where ionized matter is being ejected by a compact object, such as a black hole or neutron star. Also, from the gas in star-forming galaxies, where the high redshift is present, the very distant galaxies.
The well-known experiments such as Planck, WMAP, and COBE – all used in mapping the sky for the CMB, are examples of Microwave Astronomy, and Sub millimetre (SMA) and ALMA in Chile too.
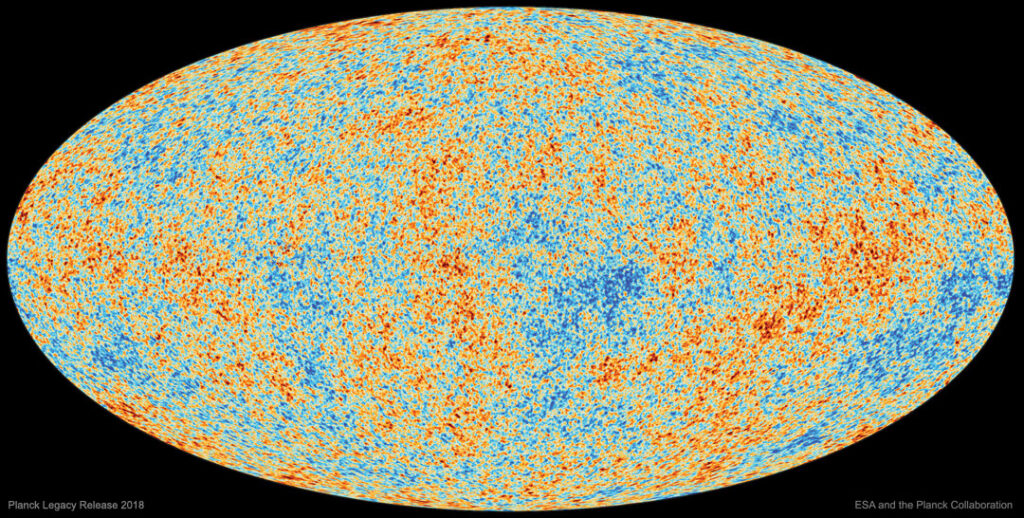
INFRARED WAVELENGTH
This spans over 15 microns to 300 microns, and a frequency of 20 THz to 1 THz. Far-infrared emission comes from thermal blackbody emission. Here, it’s due to Wien’s law – which suggests that the temperature of any object to the wavelength at which an object gives off the most light, and cool dust or gas emits far infrared. Star-forming regions, such as galaxies, or young stellar objects (i-e protostars and pre-main sequence stars), are very common and strongest sources of far infrared emission. Some examples of far-infrared missions are the Infrared Astronomical Satellite (IRAS), the Infrared Space Observatory (ISO), and the Herschel satellite.
Mid Infrared
The middle band of the infrared is also a very important source of astronomical observations, lying from the size of 2.5 microns (μm) to 15 microns (frequency of 120 THz to 20 THz), it has a shorter wavelength than far infrared light, but a longer wavelength than the near-infrared region of light. MIR is found in cosmic dust, such as it’s found around young stars, protoplanetary disks, and zodiacal dust. Near to our planet, it’s also found in objects such as asteroids, comets, and planets.
It’s a shorter wavelength than far-infrared light, but a longer wavelength than near-infrared light. MIR radiation largely traces cosmic dust, such as the dust surrounding young stars, the dust in protoplanetary disks, and zodiacal dust. The mid-infrared also traces the predominant emission of cool Solar System objects, such as planets, comets, and asteroids. NASA’s infrared Telescope Facility (IRTF), UK’s Infrared Telescope, on the ground utilizes observations from the band.
Due to the noise, and the already existing thermal background, this wavelength is very difficult to detect from Earth. So, for this purpose, the missions that are space-based such as WISE, Spitzer, and The JWST MIRI are also used for observations in this wavelength, the JWST’s MIRI has a camera and spectrograph as well, to get us a detailed view of the space objects, and their composition.
Near Infrared
It’s emitted by a hugely wide range of objects or sources, predominantly blackbody radiation, the emission from cool stars i-e M dwarfs are very good at providing Near Infrared emissions. It consists of 0.8 microns (μm) to 2.5 microns and a frequency of 380 THz to 120 THz, this wavelength can be seen from the ground-based facilities. Examples are NIR 2MASS survey, the IRTF, UKIRT, VISTA, and this is also commonly done from space-based equipment. As the JWST telescope also covers this range and it is currently changing the field of near-infrared astronomy with its instrument and position in space (L2).
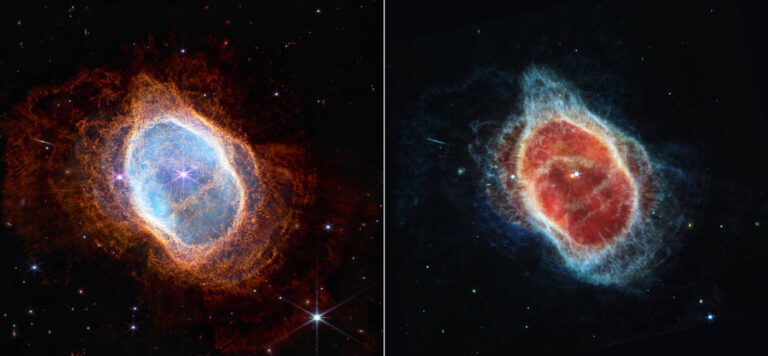
OPTICAL
This is the visible light, we see every day, the radiation for human eyes to understand and interpret, produced by blackbody processes, but also coming from non-thermal sources. Being visible from the wavelength range of 350 nm to 800nm (Frequency: 960 THz to 380 THz), the ionized gasses can also produce visible/optical light but in discrete spectral lines, not as continued sources. As our eyes can see from the ground, the visible band of the Milky Way center, and the stars in the sky with naked eyes. But, still, we have large observatories hosting optical equipment such as W.M Keck telescopes, the four VLT, and SALT. Other space-based optical observatories include the most famous Hubble Space Telescope, Kepler, GAIA, and TESS.
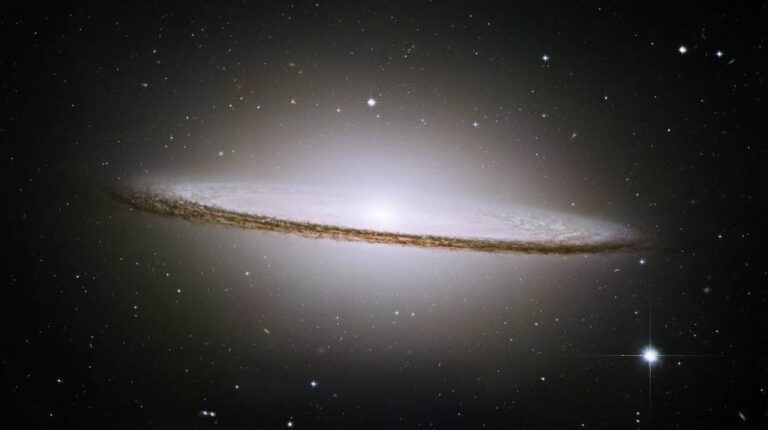
ULTRAVIOLET
This band of the spectrum is short enough to not be visible to the naked eye, it’s size can be compared to the molecules, having a wavelength of 10 nm to 350 nm and frequency of 3e16 Hz to 860nm (Energy of 120 eV to 3.5 eV). The longest ultraviolet rays are just short enough to be visible to the naked human eye. UV light can be emitted from a lot of sources and powerful non-thermal sources as well. Thermal UV comes from hot O stars and B stars as the main sequence, white dwarfs.
The non-thermal arises from AGN, our SUN i-e a main sequence star also emits UV spectra lines. But, UV cannot be observed from the ground, as most of it is blocked or extinguished by dust along the line of sight, except its longest wavelengths. Space Telescopes such as GALEX, HST, and AstroSat are very good at observing the UV band.
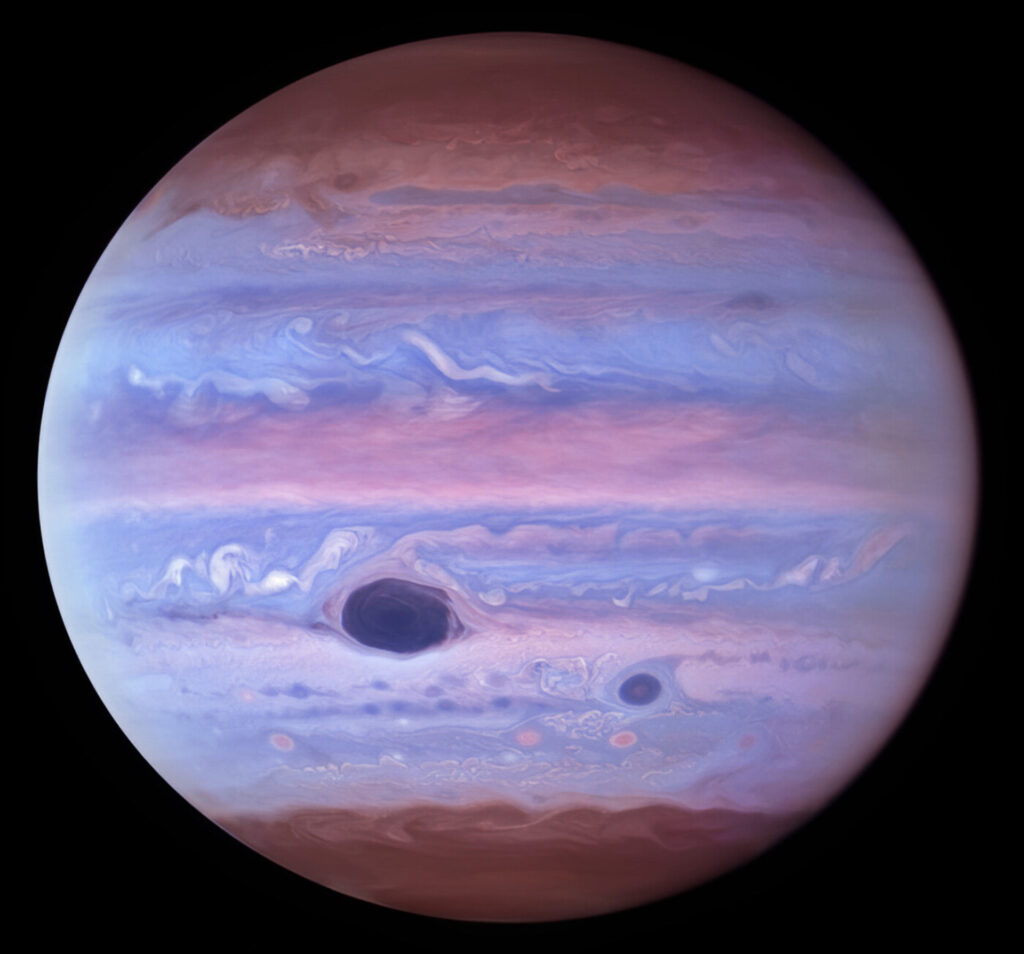
X-RAYS
X-ray emission is an emission that can be found in very hot objects which could be neutron stars or free-free emissions from hot gas clouds around the galaxy clusters. Objects that have emission, will be very compact objects, and in process of accretion – such as Black Holes, X-Ray Binaries or Active Galactic Nuclei. Well, coming onto observations, the wavelength is very short and is blocked by our Earth’s protective atmosphere, but they can surely be observed from space. Most common instruments, such as Chandra, XMM Newton, NuStar, ROSAT, Uhuru, Einstein, have collected data in X-Ray bands and made amazing discoveries in the field.
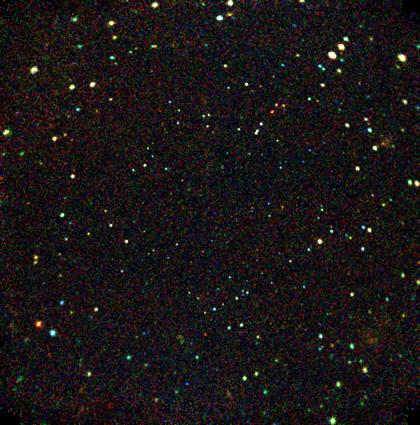
GAMMA RAY
The wavelength is the shortest in the electromagnetic spectrum; 10 pm or shorter. Its frequency would be higher than 3e19 Hz and the energy of the emission is around 120 eV or Greater. Its photons are so small that they can be compared to the size of individual atoms. This suggests that emission in nuclear physics, that gamma decay is related to it. The pair annihilation of high energy physics can also produce gamma emission.
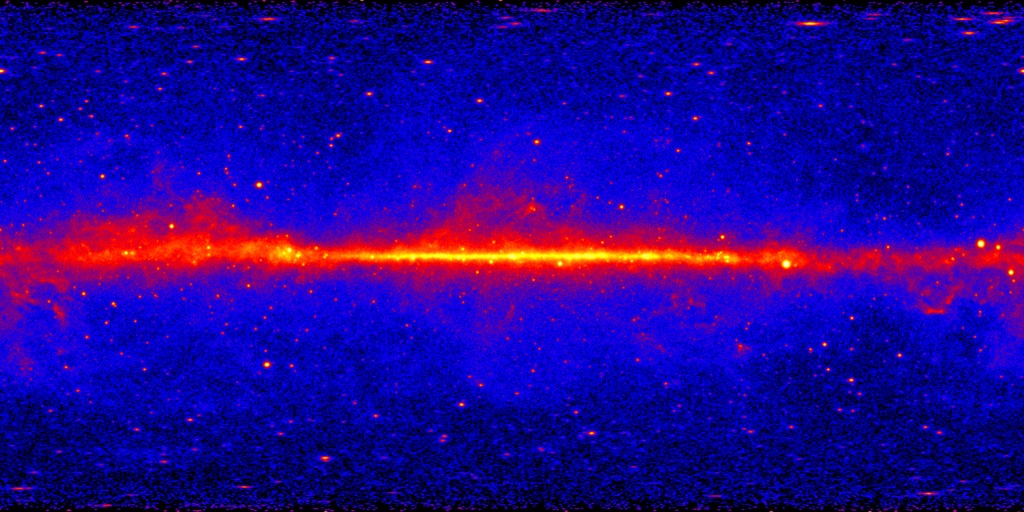
The sources of Gamma-rays in our universe are some classes of AGN, Relativistic Jets, and Compact Binaries, either also can be seen in, one of the most powerful events in the universe – Gamma Ray Bursts.
The Gamma rays can only be observed from space. For example, the Gamma telescopes are Compton Gamma-ray Observatory, the INTEGRAL, FERMI, which have contributed a lot to our understanding of this part of the Electromagnetic Spectrum.
References:
All the references are embedded as links.
The article was originally inspired by Astrobites: Guide to the Electromagnetic Spectrum in Astronomy
Also, read: The universe is astounding
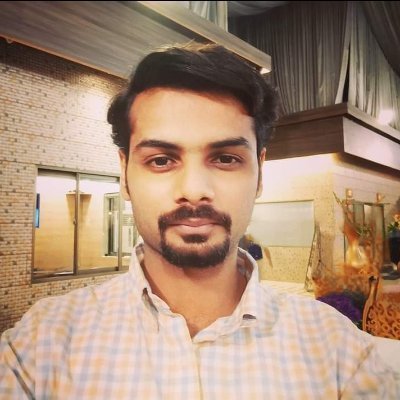
Fouz Siddiqui is a writer, academic and scientific management person. Presently, he is a Co-founder and Chief Information Officer at Scientia Magazine. As CIO, he oversees the implementation and strategization of Scientia’s technological and scientific vision. Concurrently, In academia, he holds a Lecturer and QM position at ATH – IST. As an academic, his research interests are Exoplanetary Sciences within Astronomy. Furthermore, he also works with Kainaat Studios, as its Manager of Science Outreach.